Freezing Light: The Dawn of Supersolid Photonics and Its Impact on Quantum Computing
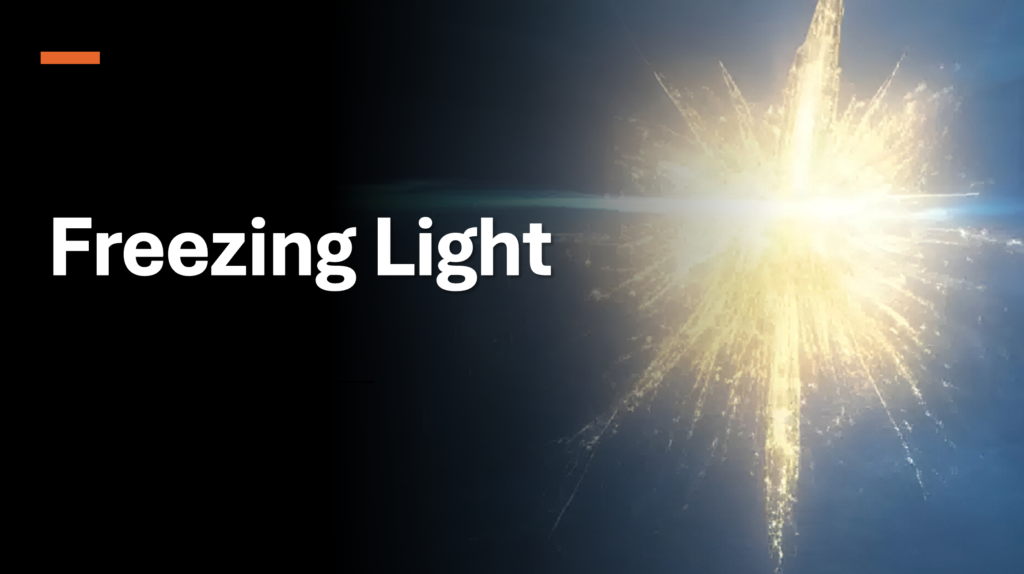
Freezing Light: The Dawn of Supersolid Photonics and Its Impact on Quantum Computing In a groundbreaking experiment, Italian researchers have succeeded in making light behave like a supersolid—a rare and paradoxical state of matter that flows without friction like a superfluid, yet retains an ordered, rigid structure like a solid. This discovery, published in Nature, marks the first time that light—long considered the ultimate free agent in physics—has been observed exhibiting both coherent motion and spatial rigidity in a semiconductor-based system. Beyond its novelty, this advancement opens profound new possibilities in quantum information science and could revolutionize the architecture of future quantum computers. Scientific Overview: Why This Matters to Quantum Technology As a quantum computing researcher, I view this development as a critical inflection point. It represents a fusion of condensed matter physics, quantum optics, and photonics, delivering a platform with properties long sought after in quantum engineering. Supersolid Light as a Medium for Quantum Information Flow Traditionally, photons are used in quantum computing as qubits, information carriers, or interconnects due to their high speed and resistance to decoherence. However, their very freedom makes them hard to trap or localize. Supersolid light changes that narrative. By creating a photonic supersolid, scientists have realized a phase where photons maintain phase coherence while holding a stable spatial distribution. This combination makes them uniquely suited for long-lived, stable quantum channels, where quantum information can be routed or entangled while maintaining spatial structure—a potential game changer for photonic quantum processors. From Bose-Einstein Condensates to Semiconductor Platforms Supersolidity had previously been limited to ultracold atom experiments under extreme cryogenic conditions. The current study shows that supersolid behavior can be engineered on photonic semiconductor chips, leveraging existing materials used in lasers, waveguides, and optoelectronics. This could accelerate the development of integrated quantum photonic circuits, bridging the gap between quantum experimental physics and scalable commercial quantum technologies. It opens the door to devices that simulate quantum materials, process quantum information, or even form the building blocks of quantum memory systems. Quantum Simulation and Supersolid Light Supersolid light represents a quantum phase of matter that can simulate exotic physical phenomena—such as phase transitions, symmetry breaking, and non-equilibrium dynamics. These are core problems in quantum simulation. Since these effects are now reproducible with photons on a chip, it’s feasible to build quantum simulators using light, rather than relying on bulky cold atom labs. This dramatically reduces costs, increases scalability, and improves compatibility with existing classical and quantum photonic systems. Toward Supersolid Quantum Devices Just as superconductivity led to practical devices like SQUIDs (superconducting quantum interference devices) and superconducting qubits, supersolid light could lead to: Supersolid photonic circuits with novel routing or phase-locking capabilities Quantum memory arrays based on stationary light modes Photonic quantum sensors that exploit the rigid coherence of the supersolid state These devices may eventually form the backbone of hybrid quantum systems, combining the best of photonic speed, atomic coherence, and chip-based integration. All in all, the ability to freeze light into a supersolid phase is not just a curiosity—it is a paradigm shift. It gives researchers a new platform to manipulate light in a way that balances freedom and structure, coherence and confinement. For quantum computing, this could mean the birth of new architectures that are faster, more stable, and more scalable. We stand at the edge of a new era in quantum photonics, where light can do more than illuminate—it can compute, store, and simulate with quantum precision. This advancement brings us one step closer to that reality. Reference: Trypogeorgos, D., Gianfrate, A., Landini, M. et al. Emerging supersolidity in photonic-crystal polariton condensates. Nature 639, 337–341 (2025). https://doi.org/10.1038/s41586-025-08616-9 Facebook Twitter LinkedIn Email